Clive Neal demonstrates the process of grinding the tiny samples of ash-like material collected from the surface of the Moon during an Apollo space mission in 1972. His graduate student, Jessika Valenciano, will prepare the minute Moon rocks from the other 19 canisters that arrived on campus in December to measure the exact chemical makeup to the parts per billion.
It’s somehow fitting that cutting-edge geological research of samples from outside this world begins with a tool that humans began using in the Stone Age. The mortar and pestle do their job so well that they may be the least-changed tool in human history. The rounded bowl and club-shaped pestle originated in the Stone Age are nearly identical to those used in kitchens today.
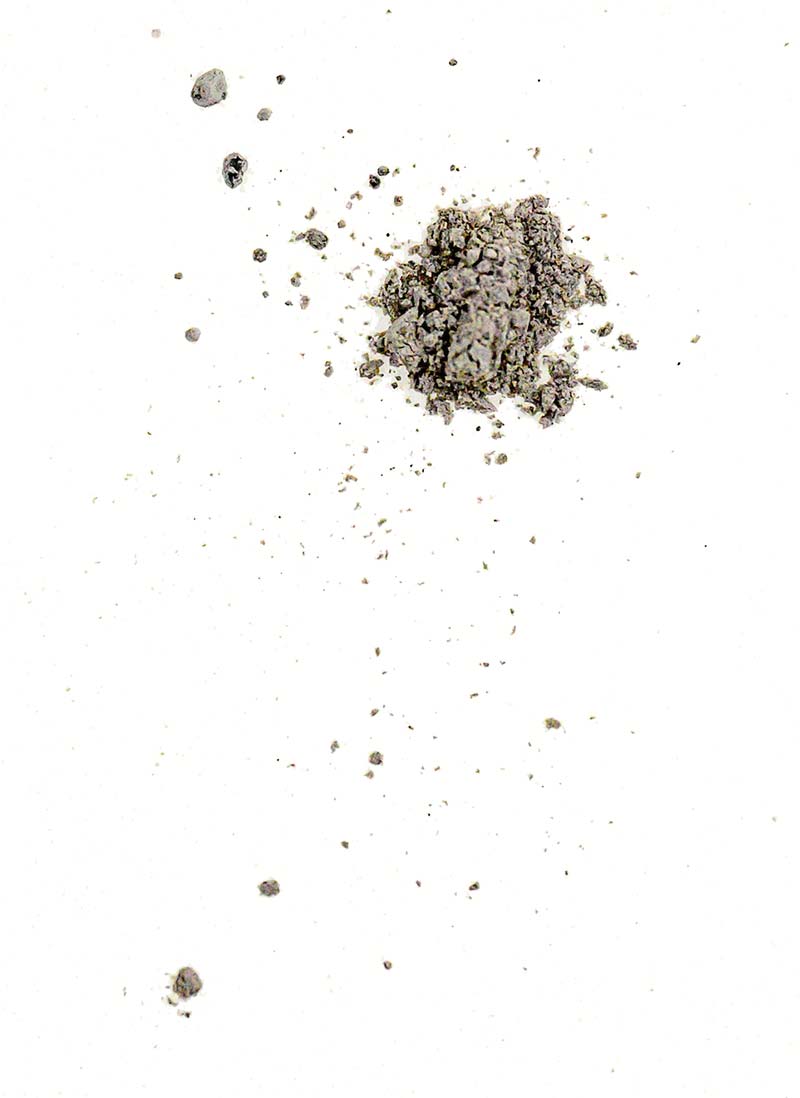
Neal, a Notre Dame professor of civil and environmental engineering and earth sciences, uses a mortar and pestle to grind 50 milligrams of lunar regolith — the ashy surface “soil” of the Moon — into a fine dust that will be dissolved in hydrofluoric and nitric acids. The solutions will be analyzed in lab machines the size of an office copier in Notre Dame’s Center for Environmental Science and Technology and Midwest Isotope and Trace Element Research Analytical Center to determine the abundances of major and trace elements the samples contain.
The samples Neal and Valenciano are analyzing were collected in 1972 from the surface of the Moon in vacuum tubes by Apollo 17 astronauts Harrison Schmitt and Gene Cernan. The tubes have remained unopened since they were returned and represent “new” samples.
“These are the first real detailed analyses from this sample — we’re starting to see a new part of the Moon that we basically haven’t seen before,” Neal said. “The samples are precious. A lot of investment went into getting them. So we’re trying to maximize the science that comes out.”
The sun continually bombards the Moon with solar wind, Neal said, because the Moon does not have an atmosphere to protect it like the Earth does. The solar wind contains a wealth of volatiles — elements like hydrogen, nitrogen, carbon and helium — that settle in the dusty regolith.
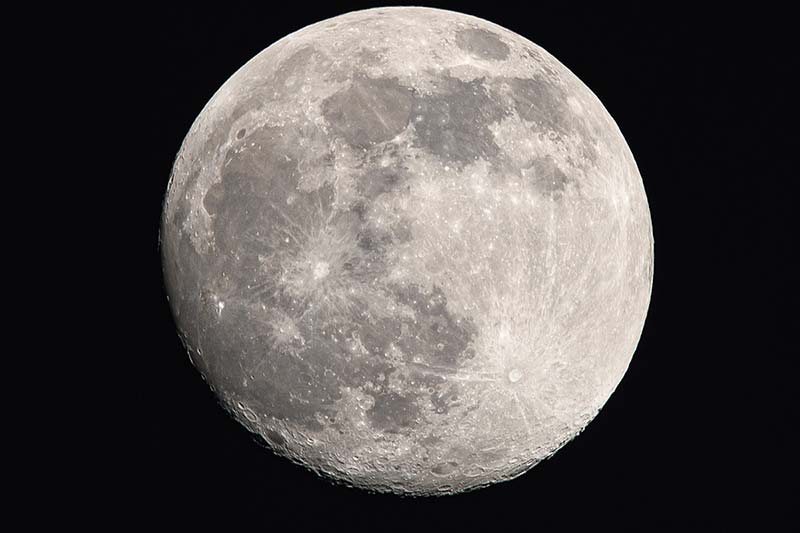
When regolith is brought back to Earth, these volatiles are lost to some degree in the process of collection and storage or in the shaking that occurs during lift off from the Moon and on re-entry to Earth’s atmosphere. What is special about the samples Neal recently received is that they were collected in a vacuum tube at Station 3, a landslide area different from the more analyzed areas where Apollo 17 landed.
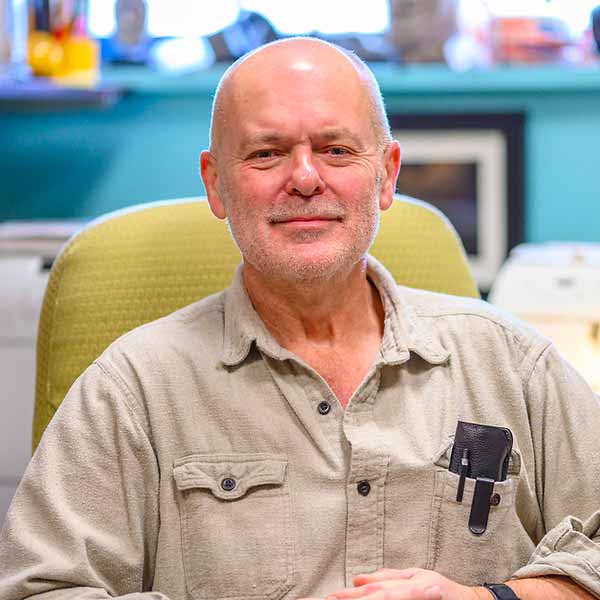
“These are the first real detailed analyses from this sample — we’re starting to see a new part of the Moon that we basically haven’t seen before.” —Clive Neal
On Earth the unopened tubes were placed in another vacuum canister for protection. For each half centimeter of depth in the canister, labs across the country and world perform different analyses. Neal received 20 vials with 50 milligrams of Moon rocks in each and expects 20 more.
“NASA just opened the canister [in December 2019], so it’s really new, exciting stuff,” Neal said. “We really don’t know what the true solar wind content of the regolith is, and therefore we don’t know the resource potential that it could have.”
Neal’s ultimate goal is to discover natural resources that will make a continual presence on the Moon economical and possibly power the next step of humans into the further reaches of space. As the former chair of NASA’s Lunar Exploration Analysis Group, he has been a consistent advocate of returning to the Moon, either as a country or as part of a consortium like the International Space Station, and likely including private companies sprouting up now.
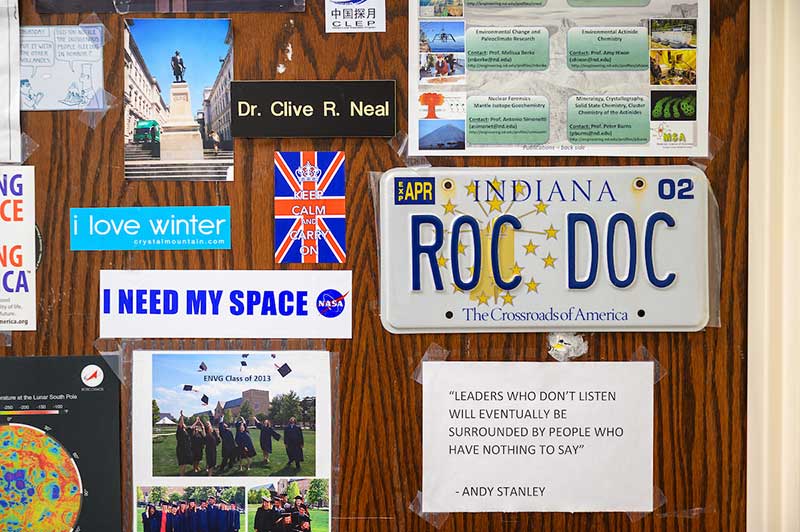
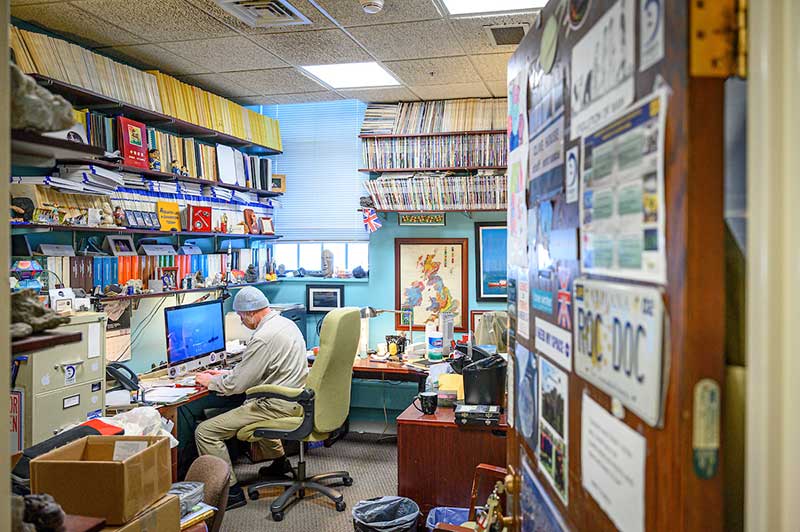
“I saw the first 12 humans walk on the Moon and now I want to see the 13th, as well as seeing humans back on the surface of the Moon to stay this time,” Neal often says.
Neal believes that America abandoned the Moon after winning the race against the Soviets to get there first with the Apollo program. He was 8 years old in Britain and remembers his father waking him to watch Buzz Aldrin and Neil Armstrong take the first walk on the Moon. He hopes his research can further our understanding of how lunar resources could support settlement and create a new economy for things like building materials, radiation protection and rocket fuel.
“We can learn from a permanent lunar settlement — how to survive and how to keep exploring,” he said. “I don’t think we will get to Mars without going to the Moon.”
Lunar samples
Rocks and regolith from the Moon are of course a rare phenomenon, one that has recently resurfaced with a Chinese mission that brought back the first samples in 44 years. The Chang’e 5 capsule landed in December with about 4 pounds of lunar dirt and gravel from a different area of the Moon than previous missions.
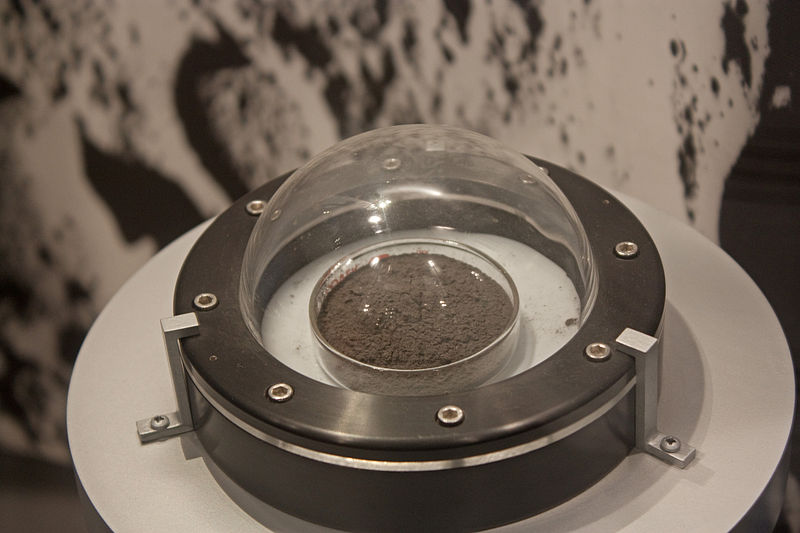
The last lunar material on Earth before December came from the Soviet Union’s Luna 24 mission, which brought back about 6 ounces of regolith in 1976. Before that, the six U.S. Apollo surface missions brought back about 842 pounds of regolith between 1969 and 1972. That haul has been Neal’s gold over the last 35 years.
The Chang’e 5 samples are expected to provide a new window into lunar history, because they come from a region of the Moon believed to have formed about 1.5 billion years ago. The volcanic rocks Apollo brought back were older than 3 billion years.
“We know they landed on a terrain that’s much younger than the Apollo or Soviet missions,” Neal said. “One and a half billion years is young for the Moon. Relative age dating has been carried out by looking at the number of craters in a given surface and comparing those with Apollo, where we have samples.
“These Chang’e samples are completely different from what we have and are an exciting new chapter in our understanding of the Moon.”
While Neal would love to study their samples, he said the Chinese government recently published requirements on how to apply for access. Even though Chinese scientists can’t apply for Apollo samples, Neal hopes to study the Chang’e 5 samples through a consortium of scientists, a model he thinks is necessary to research and fund modern space exploration.
“There’s an international group that I’m part of that is hoping to get involved with the Chinese samples, without any NASA funds,” he said.
Scientific precision
The rarity of lunar material explains why the process of analyzing them is so meticulous. It starts in a Cushing Hall lab near Neal’s office by opening each numbered vial and weighing it exactly. The clean lab requires removing shoes and wearing white lab coats and safety glasses.
A vacuum-sealed plastic bag contains smaller bags of labeled metal vials. The dusty regolith weighs slightly less than 50 milligrams because some sticks on the surface of the vial and lid. It gets poured into a small mortar bowl, which has a cover with a hole in it so that the pestle can grind it without losing dust into the air.
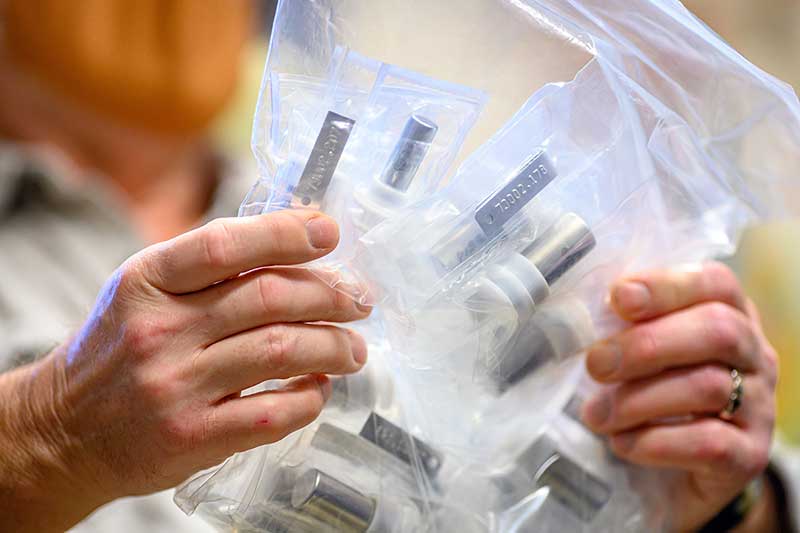
Neal shows Valenciano the grinding method and lets her take over. The results of this research will become her doctoral dissertation.
“We want to get it to a talcum powder consistency,” he explains. “This is critical because we can’t regrind after it goes in the acid — we need complete dissolution.”
The Apollo regolith weighs slightly less again after grinding, as a small amount sticks in the mortar. It is put in a small plastic vial to await dissolution in hydrofluoric and nitric acids. The mortar and pestle get wiped down with dilute nitric acid and ultrapure water to avoid cross-contamination with the next sample to grind. Valenciano adds the acids to each sample when five vials have been properly prepared.
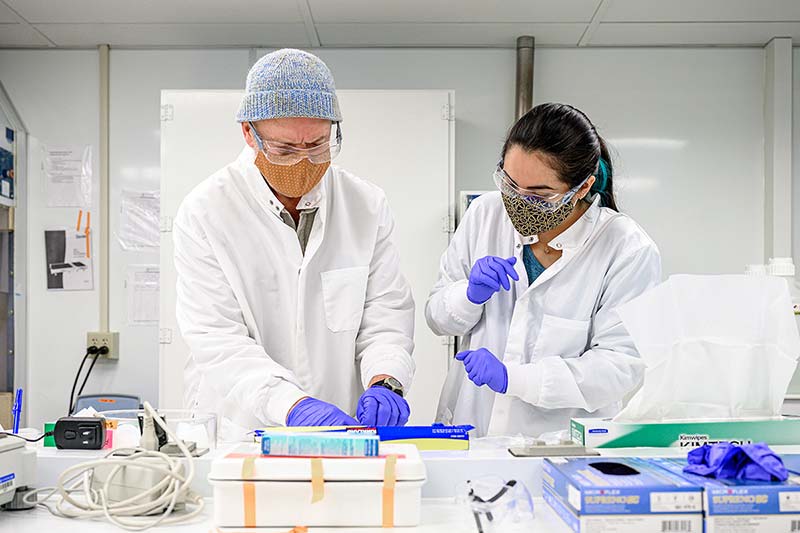
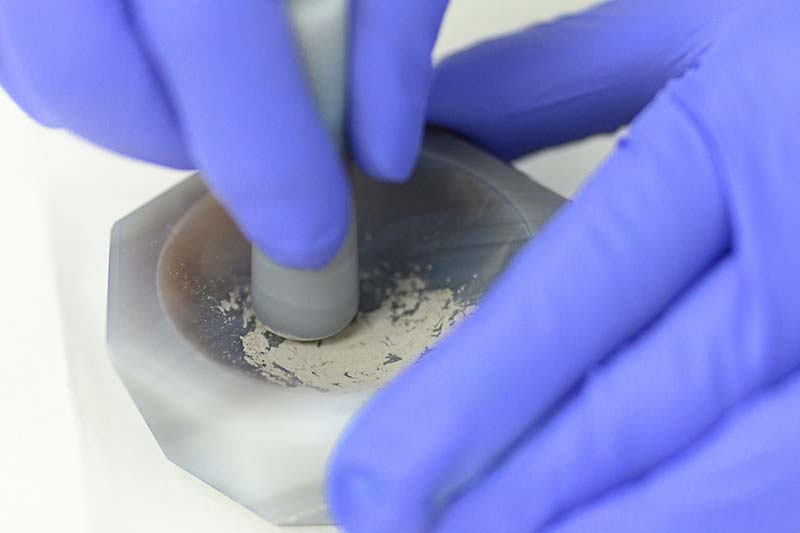
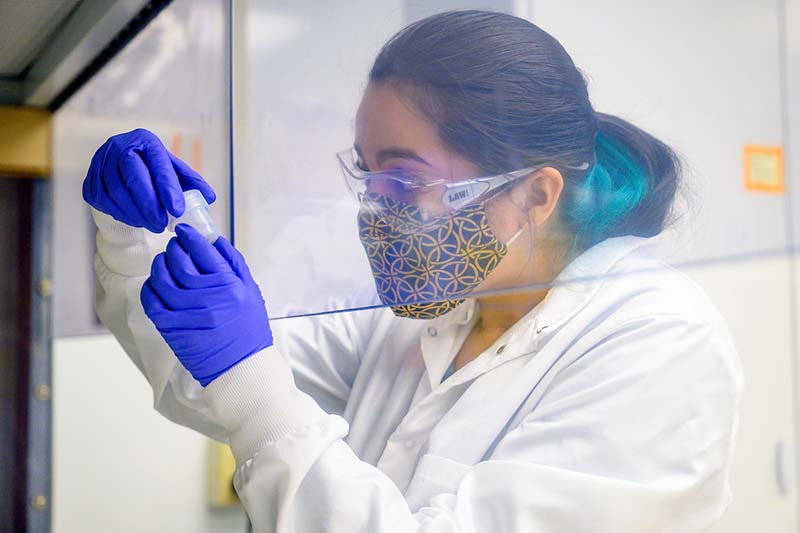
The dissolved samples are now ready for the analyses that will determine the major and trace elements down the sample tubes, what Neal calls the “chemical stratigraphy.”
“We have two separate machines, both based on inductively coupled plasmas (ICP),” he said. “Basically, you use a radio frequency to induce a plasma that atomizes and ionizes the liquid at high temperature. Then the ICP emission spectrometer looks at the plasma, and the strength of the bands in the spectra get you a full quantitative major element analysis. The ICP mass spectrometer counts ions and gives a full quantitative trace element analysis”
Over 30 years at Notre Dame analyzing lunar and asteroid material, Neal has advanced human understanding of the Moon’s geology and history. His teams have performed the first comprehensive study of the full range of trace elements, identified minerals from different source regions and made some of the first full analysis of volcanic basalt rocks.
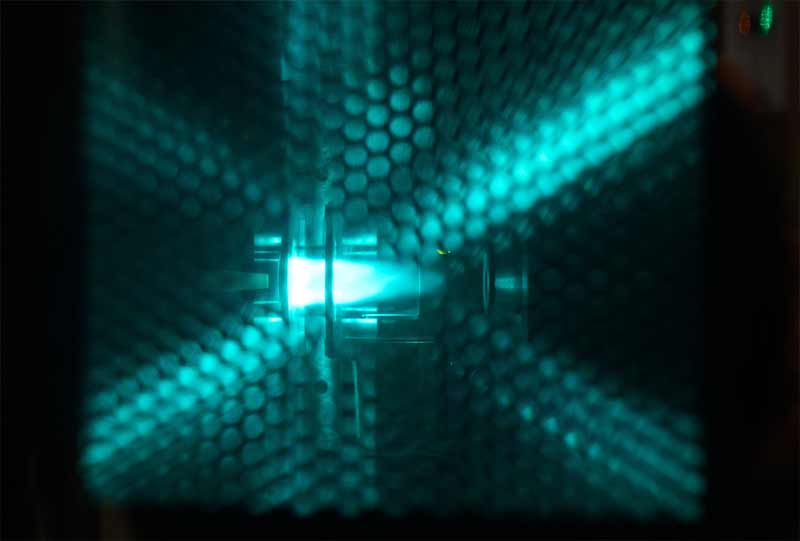
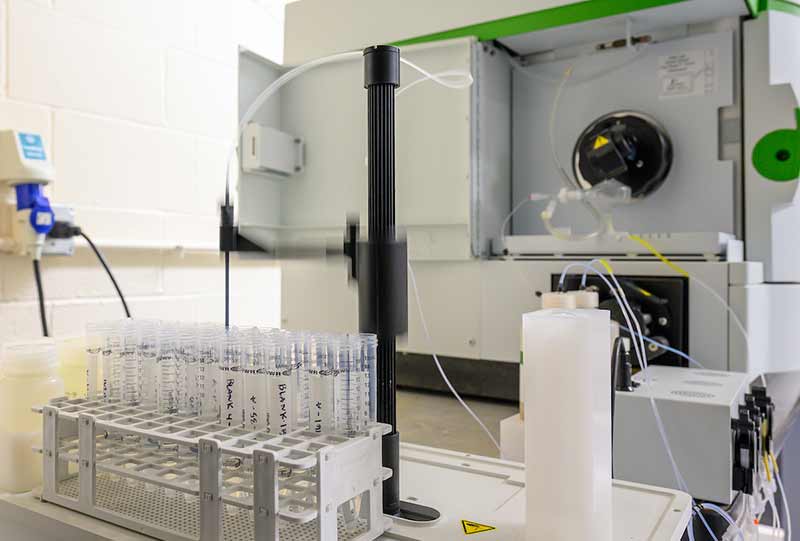
His process is destructive, meaning that the sample is used up, but other portions of each half-centimeter in the vacuum core tube will remain. Other labs, such as the University of New Mexico and Washington University in St. Louis, are performing different analyses on the same samples.
“It takes an international consortium to do this because no one lab has all the instrumentation you need,” Neal said. “That’s why you bring together different people with different expertise.”
Shoot for the Moon
While the precious regolith might look like the ash left in your fireplace, it does come from another celestial body and therefore has very different properties. One of the volatiles that solar wind implants in the lunar dust is hydrogen.
“I’ve seen this experiment done at Johnson Space Center where you take lunar regolith, put it in a vacuum, heat it up and water drips out the bottom,” Neal said. “Now, there’s no water in there, but it is exciting those hydrogen atoms to then combine with oxygen in iron oxides to create metallic iron and water.”
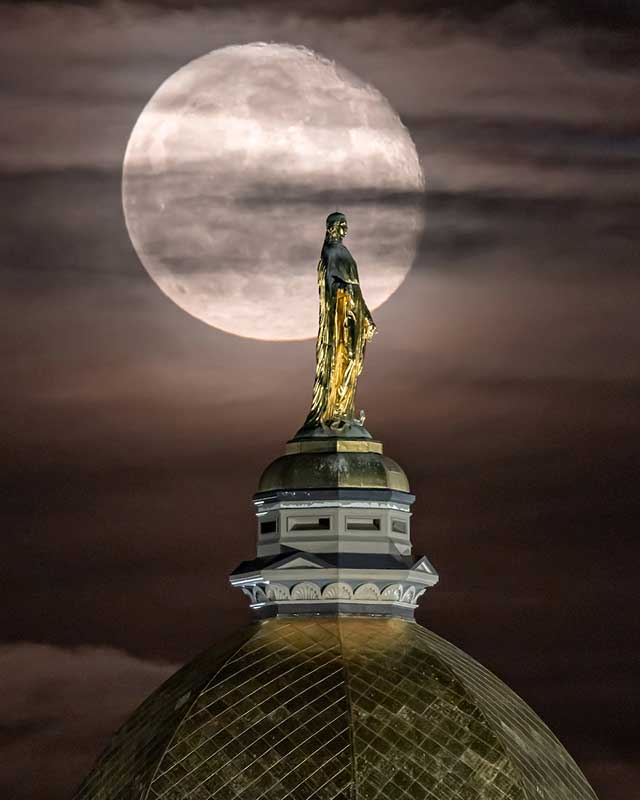
This kind of scientific wonder shows why Neal believes a permanent settlement on the Moon is more than science fiction. But why would humans want to spend exorbitant and limited capital to go to a place we’ve already been? What reason would induce us to spend the necessary money and effort?
Neal argues that space exploration pays great dividends, and not just by sating our curiosity. Many of the inventions necessary for survival in space have advanced the science and engineering of products that benefit us at home, such as the cellphone.
If humans are going to go further in space, he believes the necessary science and technology will be invented through humans surviving and thriving on the Moon. His final argument shows how that promise could eventually be realized.
Helium-3 is an extremely rare isotope on Earth. But scientists like Neal have shown it is relatively abundant on the Moon as another volatile that solar wind embeds in lunar regolith. If helium-3 could be mined on the Moon, then scientists theorize that it could be used as material for sustained nuclear fusion, a technology that China and other countries are now developing.
“It’s the nirvana of nuclear fusion,” Neal said. “There’s no toxic byproduct. Theoretically, one shuttle cargo bay of helium-3 from the Moon, which is mining about two to three football fields down to a meter or a meter and a half, from what we know from Apollo — and you fuel the entire United States for one year.”